Citizen Science #20 by Jamie Zvirzdin
Energy Demystified: Thermal Energy, a Hotbed of Activity
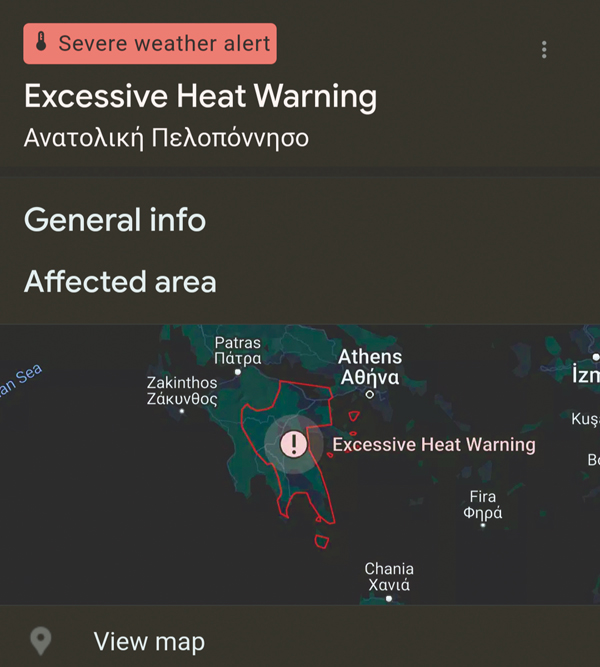
Every Sunday afternoon during the COVID-19 lockdowns, my husband and I met up with our neighbors, Beth and Charlie, for drinks in their back yard. We set up lawn chairs six feet apart and talked about anything and everything in the humid Maryland heat. The tradition continued through the freezing winter, as we donned extra layers of clothing and grasped steaming mugs of wassail and hot toddies. The next year, as sweat again slipped down our necks and condensation off our beers, we made a plan to check an item off Charlie’s bucket list: to go sailing in Greece in August 2024.
Thermal energy was absolutely not on my mind when we made these plans.
In 95-degree temperatures and through Google-issued heat warnings, our sailboat heaved to and fro across the Aegean Sea. On more than one occasion, so did my lunch. We made it back safely and had a marvelous time together, but I learned three things: a) how much I love Greece’s language, history, and food; b) how little our word “nausea” has changed from the original Greek word “nausía” (ναυσία, seasickness); and c) how quickly heat rash and heat exhaustion take the wind out of my sails. Despite how poorly I reacted to both motion and heat, I am still fascinated by how both motion and heat are so intricately connected to thermal energy.
In the world of thermal physics, energy takes on multiple names. Each plays a role in shaping the behavior of matter, and each helps describe specific physical situations. Physicists still argue over naming conventions, but the main players are internal energy (U), work (W), heat (Q), Gibbs free energy (G), Helmholtz free energy (F), enthalpy (H), and calories (kcal). Temperature itself is proportional to the average kinetic energy of the particles in a system, an energy of motion also measured in Joules.
These names, symbols, formulas, and applications may differ, but underneath it all, from a dimensional perspective, they all stem from the same physical quantity: energy. That is, they can all be measured in units of Joules, just like the other flavors of energy we’ve studied this year. Knowing this can help you sort the multilingual alphabet soup that is physics.
Given that Greece, motion, and heat have been so very interconnected for me this summer, let’s nail down these concepts using analogies from Greek mythology. We’ll concentrate on just the first three concepts here, although I hope you’ll explore the others if you like this particular form of energy.
First, internal energy (U) is the sum of all forms of microscopic energy in a system. It includes all the kinetic energy (the motions, rotations, and vibrations) of tiny particles and all the potential energy of those particles interacting with each other. Think of Pandora’s box (in the original myth, it’s a jar, not a box!) rollicking with buzzing, sparking, invisible particles of evil and mischief inside. “Thermal energy” is a term often used interchangeably with internal energy, but generally, it’s the energy that comes from random molecular motion. We can’t ever know the total internal energy exactly, but we can study what happens when there’s a change in the system.
When Pandora opened the jar—changed the state of the system—the evil emerged, changing the state of the world. The jar still contained hope, however, a gift to Pandora from the gods. When it comes to internal energy, we can’t ever measure what’s in that jar exactly before or after Pandora opens it, just as we can’t measure evil or hope, but we can definitely understand what changes.
We’ve already talked about kinetic and potential energies this year in our “Energy Demystified” series, along with work (W), but work is so important in thermal physics that it deserves its own analogy. I like to think of work as Hephaestus—god of fire, volcanoes, metalworking, and craftsmanship—banging around his forge, swearing, and sweating. Just as Hephaestus uses his hammer and anvil to forge weapons for the gods, work in thermal physics is the energy transferred when we apply force to move objects or change states.
Hephaestus can do work on a system, like hammering on a glowing-hot sword, applying an outside force to shape it, just as external pressure does work on a gas, compressing it and changing its volume. In both cases, energy is transferred from the outside—the hammer or the pressure—into the system, transforming the state of the sword. This kind of work shows up as a positive quantity in thermal physics equations.
Alternatively, work can be done by a system; think of Achilles using this finished sword to ruthlessly skewer enemies. In this case, the sword (the system) is doing work on its surroundings, converting its stored potential energy into mechanical work. Just as a gas (like steam) expands and pushes against a piston, doing work on its environment, so too does the sword apply force over a distance, transforming its potential into action. This shows up as a negative quantity in equations, as we’ll see in a moment.
Heat energy (Q), finally, can be envisioned as the warm hearth fire tended by Hestia, the virgin goddess of the hearth, home, and sacred and sacrificial fires (also pigs). In this other kind of forge, heat is the energy transferred because of a temperature difference, and it flows naturally from hotter to cooler objects, just as Hestia’s fire spreads warmth throughout her kitchen on Mount Olympus.
Hestia’s cooking is all about this flow: She adds heat to her pots and pans, transforming raw ingredients into meals, just as heat is added to a system to raise its temperature or cause a phase change. When she removes a pot from the fire, the heat leaves the system, and the contents cool down, just as heat can be subtracted from a system, lowering its total internal energy state.
This flow of heat energy, Q, into or out of a system, is one of the central ideas in the First Law of Thermodynamics, which essentially states that energy cannot be created or destroyed, only transferred or transformed. Picture Hestia adding heat to the system, a pot of water: as she stokes the flames under the stew pot, the total internal energy of the system increases, either raising the temperature of the contents or causing a change in state (the water boils, starts steaming). In turn, the steam pushes against the lid of the stew pot, creating work done by the system (a negative quantity, like waving around a sword): The steam starts escaping from underneath the lid. The mathematical expression of this is the equation for the First Law of Thermodynamics:
dU = δQ − δW,
which means “a tiny change in the internal energy equals a different kind of tiny change in heat minus a tiny change in work done by a system on its surroundings.” If Hestia removes the pot from the flames, energy flows out of the system as heat is transferred from the hot pot to the cooler air.
Alternatively, what if Hestia decides, unwisely, to do work on the system—like Hephaestus hammering on the sword—by forcing the lid down tightly on the pot, even as the temperature inside the pot increases? In this case, she is doing work on the pot of water, driving up the internal energy to a dangerous degree. The volume of the pot remains the same, but since the temperature is going up, pressure must also go up. This is the essence of the pressure cooker. When work is done on the system, our internal energy equation becomes
dU = δQ + δW,
and Hestia should really reconsider her cooking methods.
There’s so much more to thermal physics—“thermal” itself comes from the Greek adjective “thermós” (θερμός), meaning hot or warm—so the next time you sip a hot or cold drink, perhaps in the company of your neighbors, think of the godlike forces at play around you contributing to the experience. If you travel, prepare for unexpected thermal shifts; climate change is, unfortunately, not a myth. Just as we were leaving Athens, in fact, a major wildfire moving at lightning speed broke out to the northeast, the worst Greece has seen this year.
As our world’s internal energy increases, be ready to confront, understand, and embrace the science of thermal energy. The laws of thermodynamics aren’t going anywhere, so as things heat up, let us be the change that brings equilibrium to our system.
Jamie Zvirzdin researches cosmic rays with the Telescope Array Project, teaches science writing at Johns Hopkins University and is the author of “Subatomic Writing.”